Chapter 13. Developmental Genetics
Human Reproduction and Development
All mammals, including humans, reproduce sexually.
The human reproductive system consists of a pair of gonads (ovaries or testes) where the gametes are produced, a system of ducts that house and conduct the gametes, and structures that facilitate copulation.
The human female has a pair of ovaries that contain egg cells (oocytes) surrounded by nourishing cells. An egg cell surrounded by these supportive cells is called a follicle. An egg cell is released from the follicle during ovulation into an oviduct (fallopian tube).
The uterus (the womb) houses the embryo (0 – 9 weeks) and the fetus (9th week – birth) during pregnancy.
The male reproductive system consists of a pair of testes that produce sperm; a system of ducts that moves sperm out of testes, and a set of glands that produce supportive fluids. Sperm mixed with the secretions of the glands forms semen that is discharged during ejaculation.
The formation of gametes (sperm and ova) requires meiosis.
Spermatogenesis is the formation of sperm cells.
The first division of spermatogenesis involves the production of haploid secondary spermatocytes from diploid primary spermatocytes.
Haploid sperm cells form during the second division of spermatogenesis.
Oogenesis is the formation of an egg (ovum).
Oogenesis begins prior to birth. At birth, follicles contain diploid primary oocytes (arrested at the tetrade stage of Prophase I of Meiosis I).
At puberty, a single primary oocyte completes Meiosis I each month, producing a haploid secondary oocyte and a polar body (smaller cell, not essential in reproduction) and progresses to Metaphase II of Meiosis II.
The second division of oogenesis takes place when a sperm cell penetrates the secondary oocyte, and yields a haploid ovum and a second polar body.
Fertilization stimulates the ovum to complete the meiotic division.
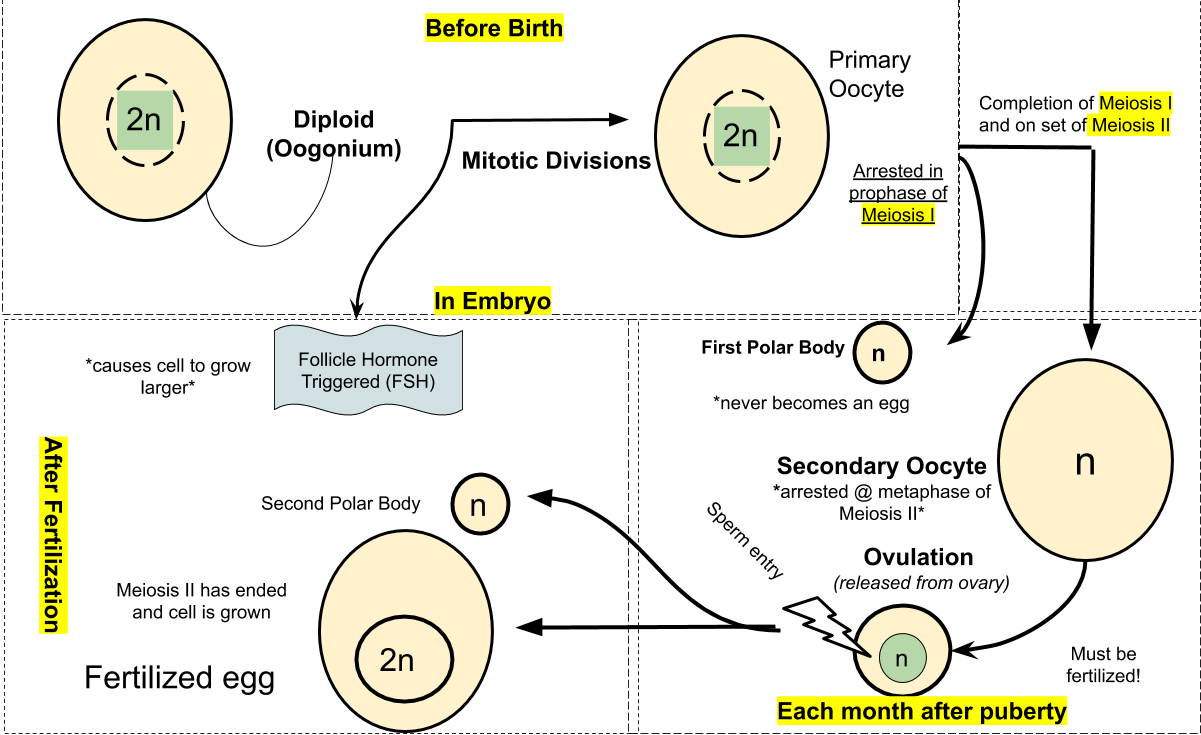
Human development from conception to birth is divided into three trimesters (three months each). Childbirth is hormonally induced and occurs in three stages.
Stages of early human development: fertilization, cleavage, gastrulation.
During fertilization, the haploid nucleus of the ovum fuses with the haploid nucleus of the sperm cell, producing a zygote.
Fertilization: the union of egg and sperm:
- occurs in fallopian tubes (oviducts);
- out of 200 million sperm released per ejaculation, only one sperm penetrates the zona pellucida (clear zone around egg); upon this event, cortical granules are released that prevent any other sperm from penetrating the egg;
- after fertilization, the egg and sperm nuclei fuse, and a new diploid human zygote is formed.
After fertilization, the zygote undergoes a series of cell divisions (cleavage), producing a multicellular embryo. Cleavage partitions the embryo into developing regions. Cleavage produces A blastocyst, which contains a group of differentiated cells on the outer surface (the trophoblast cell layer) and still undifferentiated inner cell mass.
Day 1 post-conception: first cleavage – one cell becomes two. Day 2: second cleavage – 4-cell stage.
Day 3: 6-12 cell stage – can test at this stage for genetic diseases by Preimplantation Genetic Diagnosis, PGD (if fertilization done in vitro (IVF)).
Day 4: 16-32 cell stage – solid ball of cells – morula.
Day 5: Solid morula develops into hollow, fluid-filled blastula.
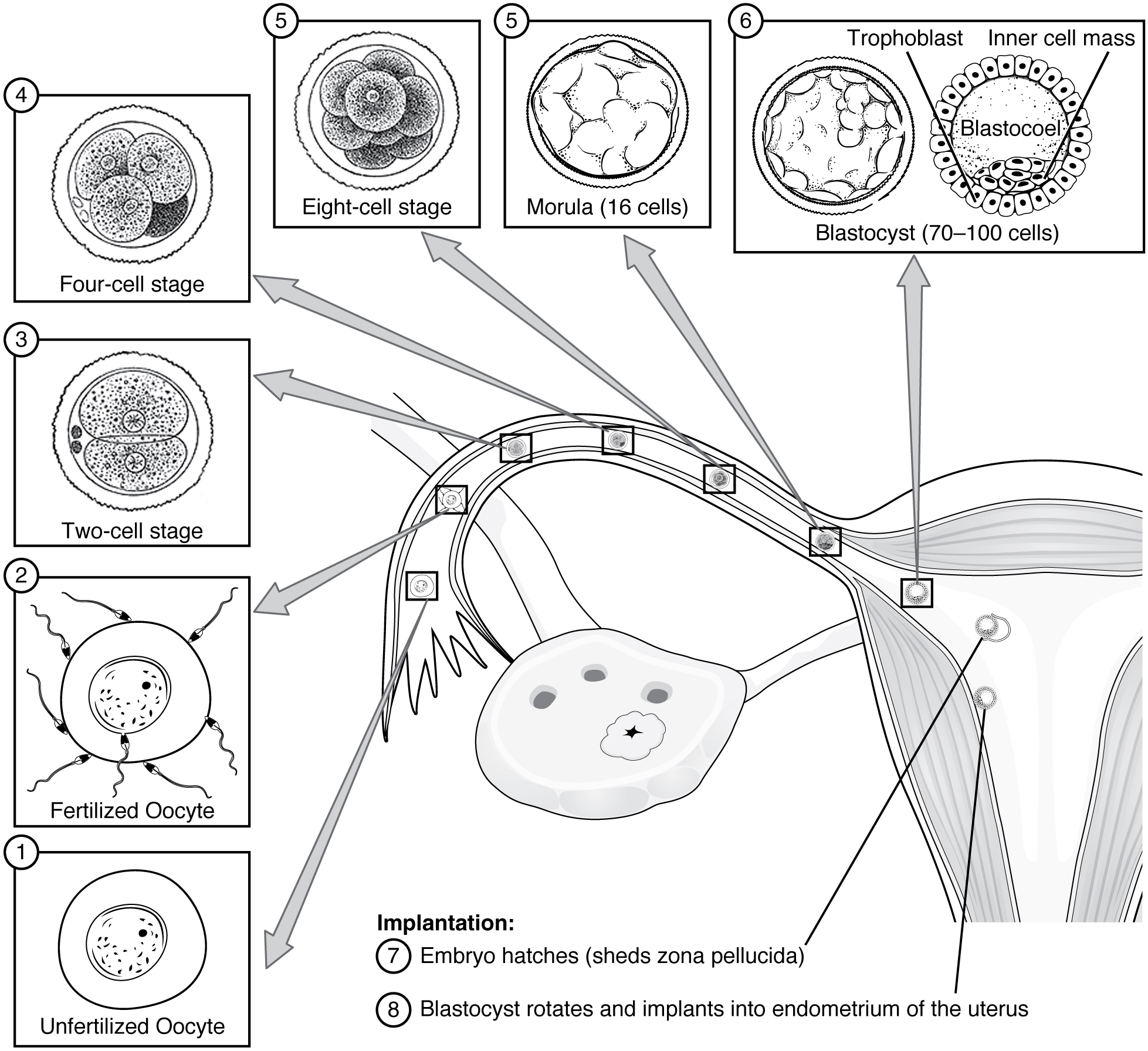
The blastocyst consists of under-differentiated inner mass cells surrounded by terminally differentiated cells of the trophoblast. Inner mass cells are the source of embryonic pluripotent stem cells, which, upon proper stimulation, can become most cells of the body. Stem cells are discussed in detail in Chapter 14.
The blastocyst implants in the uterine wall lining by about 7th day after fertilization. Implantation is aided by the enzymes released by the trophoblast cells. At the time of implantation, the upper layer of the inner cell mass forms a separate group of cells called an epiblast, the lower (outer) layer is called the hypoblast.
Gastrulation occurs by the inward movement of epiblast cells and forms the three tissue layers of the embryo: ectoderm, endoderm, and mesoderm. At later developmental stages, the ectoderm produces skin tissue and nervous system, the endoderm produces the lining of the digestive and respiratory tracts, and the mesoderm forms most other organs and tissues (heart, muscle, bone etc.).
Cells of the trophoblast give rise to chorion (the embryonic portion of the placenta); the hypoblast cells form the yolk sac (contains no yolk) where early blood cells form and later migrate to the embryo proper.
Day 6 –7, implantation: Blastocyst attaches to the endometrium and burrows in.
Days 10 – 14: Pregnancy becomes established:
- Fluid filled amniotic cavity starts to form;
- Yolk sac starts to form (will make blood cells, germ cells); -! Embryo starts to form from embryonic disc;
- Chorion (placenta) starts to form.
Developmental processes that take place after gastrulation:
Organ formation:
neural tube (future spinal cord and brain) and heart are formed by the 26th day of embryonic development;
pattern formation (the shaping of an animal’s major body structures).
The embryo and placenta take shape during the first month of pregnancy.
Week 3 – Week 8 (Embryo) – Development of all organ systems
Days 15 – 21: Emergence of the vertebrate body plan:
- Primitive streak starts to form – this is the site of gastrulation (formation of the 3 tissue layers – ecto-, endo-, and mesoderm);
- Neural groove (future spinal cord and brain) begins to form;
- Somites (bands of tissue that will become muscles and bones) begin to form;
- Pharangeal arches (future face, neck, mouth, nose) begin to form.
Day 22: Cardiac cells (early heart) begins to beat.
Week 9 – Week 40: (Fetus):
After ~12 weeks, the organ and pattern formation is largely “finished” – except brain and lung development.
Gene Regulation During Eukaryotic Cell Differentiation And Development
Embryonic development depends on genetic as well as environmental influences that are temporally and spatially adjusted to one another. The factors that, for example, determine the interactions between the tissues, the migration and differentiation of the cells, the proliferation of the cell colonies, as well as the apoptosis (programmed death of cells) are numerous. Embryonic development is a process of growth and differentiation in which the embryo becomes increasingly complex and is more and more enhanced with structures and functions. The growth depends on the somatic multiplication of the cells through mitosis. One of the most fascinating aspects of embryonic development is the fact that out of a simple zygote (fertilized oocyte) an organism arises that consists of billions of cells.
A human body consists of about 250 cell types, each with a different morphology, biochemistry and role they play in the organism, while each cell type (except for reproductive cells) are genetically equivalent, i.e., they all have the same amount of DNA. The distinctions between cell types are the result of differences in gene expression patterns. Thus, somatic cells differ in structure and function not because they contain different genes, but because they express different portions of a common genome.
Some eukaryotic genes are only expressed during development. Most eukaryotes (and some bacteria) switch genes off and on at different stages in their development. Developmental regulation (also called the long-term regulation of gene expression) enables the biochemistry of an organism to be altered in response to the particular requirements of each stage of its life cycle.
Master control genes
The human body is composed of several trillion cells. All of them originate from the same single fertilized egg, yet almost all of them have become specialized to perform certain tasks. But if all of the body’s cells (except for the lymphocytes) contain the same genes, how does one cell become a nerve cell rather than, say, a liver cell? The answer lies in the fact that not all the genes in a cell are activated at the same time. Some proteins in the nuclei of cells will, by binding to their DNA, trigger the expression of only certain genes of this DNA. And products of these particular genes will be proteins whose function will contribute to making the cell more specialized.
MyoD and muscle cell differentiation
Muscle cells develop from embryonic precursors that have the potential to become a number of alternative cell types, including cartilage cells, fat cells, or multinucleate muscle cells. As the muscle cells differentiate, they become myoblasts and begin to synthesize muscle-specific proteins. They fuse to form mature, elongated, multinucleate skeletal muscle cells.
Researchers developed a hypothesis that certain muscle-specific regulatory genes are active in myoblasts, leading to muscle cell determination. To test this, researchers isolated mRNA from cultured myoblasts and used reverse transcriptase to produce genomic DNA complementary to isolated RNA (called complementary DNA or cDNA) containing all the genes that are expressed in cultured myoblasts. Transplanting these cloned genes into embryonic precursor cells led to the identification of several “master regulatory genes” that, when transcribed and translated, commit the cells to become skeletal muscle.
One of these master regulatory genes is called myoD. The myoD gene encodes MyoD protein, a transcription factor that binds to specific control elements and stimulates the transcription of various genes, including some that encode for other muscle-specific transcription factors. These secondary transcription factors activate the muscle protein genes.
MyoD also stimulates expression of the myoD gene itself, perpetuating its effect in maintaining the cell’s differentiated state. MyoD protein is capable of changing fully differentiated non-muscle cells into muscle cells. However, not all cells will transform. Non-transforming cells may lack a combination of regulatory proteins, in addition to MyoD.
Certain eukaryotic genes are expressed in a sequence during development. A master control gene is the first gene activated in a hierarchy that leads to differentiation along a particular pathway. Master control genes encode the first transcription factor in a hierarchy. A master control gene product activates the next set of genes that encodes the next set of transcription factors, and the cascade of gene expression has been set in motion.

This cascade of gene expression starts at first mitotic division of a developing zygote and is controlled by cytoplasmic determinants in the oocyte. This type of control is called autonomous regulation. Examples of such determinants are maternal effect genes (rather, the RNA copies of those genes).
Maternal effect genes
Maternal factors govern early embryonic development before embryonic genome activation takes place. Maternal effect genes (MEGs) are required for early embryonic development. MEGs are transcribed during oogenesis and their mRNA remains in the oocyte through the early cleavage stages of the developing embryo. In addition to MEG’s mtDNA, maternal genetic determinants may include other factors, but their biochemical nature remains poorly understood.
Maternal genetic determinants are distributed asymmetrically in the cytoplasm of the oocyte, which leads to unequal distribution among cells after cell division, subsequently affecting the activity of genes these maternal determinants are designed to regulate. The maternal genetic determinants in the oocyte influence the course of early development by producing a set of proteins (transcription factors) that initiate the transcription of the genes of the developing embryo. A subsequent cascade of gene activations sets up the developmental process that gives rise to the various parts of the organism (morphogenesis).
Most of the maternal effect genes are known from non-mammalian organisms. The presence and importance of maternal effect genes in early mammalian development has been only inferred until the recent identification of maternal effect genes in mice, such as Mater and Hsf1. A human homologue of Mater has been identified as well. To date, little is known about the mechanisms by which maternal products direct early development in mammals.
Homeobox genes
Some of the most essential embryonic genes are called homeo- box genes. Every organism has a unique body pattern because of the influence of homeobox genes. These specify how different areas of the body develop their individual structures (arms, legs etc.).
Homeotic genes are regulatory genes that determine where certain anatomical structures, such as appendages, will develop in an organism during morphogenesis. Homeotic genes encode homeotic proteins that function as transcription factors that switch on other genes. The homeobox is a sequence within a homeotic gene that contains 180 nucleotide-long sequences, which code for a DNA-binding domain (homeodomain).
In humans, the homeobox gene family contains an estimated 235 functional genes. Homeobox genes are present on every human chromosome, and they often appear in clusters. Mutations in homeobox genes are responsible for a variety of developmental disorders (craniofacial abnormalities etc.). Some homeobox genes act as tumor suppressors, which means they help prevent cells from growing and dividing too rapidly or in an uncontrolled way. For example, mutations in the HOXB13 gene can lead to prostate cancer.
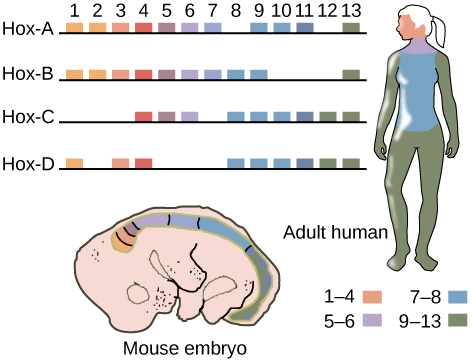
Cell-to-cell signaling and transduction pathway during embryonic development
Adjacent cells in the developing embryo are able to send sig- nals to each other (cell-to-cell signaling). This signaling allows the cells to determine their fates during development. Such cellular induction is another major mechanism that regulates embryonic development.
The induction signal can be a diffusible molecule that is secreted by the inductor and binds to the surface receptor on the reacting cell. The signal molecule can also travel along the extracellular matrix and bind to the reacting cell. Induction may also take place via direct cellular contact, during which the signal molecule leaves the inductor and enters the reactant cell via a gap junction.
Cells in the developing organism also communicate remotely through the signal- transduction pathways. A signal- transduction pathway is a series of molecular changes that convert a signal received by a target cell’s surface into a specific response inside the cell.
Genes that perform key functions during development are conserved among the eukaryotic organisms. For example, the genes involved in the development of the Drosophila, with few exceptions, are the same as those involved in the development of higher organisms. Developmental biology studies the sequential activation and interaction of genes, in relation to developing morphology.
Cell differentiation can be reversed. The nuclei of most differentiated animal cells have all the genes required for making an entire organism. Animal cloning is a procedure that involves the replacement of the nucleus from an animal egg cell with the nucleus from a somatic cell of another animal of the same species, and an induction (chemical and physical) of cell division in the modified egg.
Cell differentiation begins at early stages of embryonic development. A mammalian embryo that underwent several stages of cell division after fertilization consists of a ball of cells called blastocyst. The inner cell mass cells of the blastocyst can be extracted and grown on a nutrient medium outside of the blastocyst. Such cells will have a potential to become any cell of the body (liver, muscle, heart etc.). These cells are called embryonic stem cells. Stem cells are sometimes found in adult tissues and organs. Stem cells will be discussed in more detail in Chapter 14.
Key Takeaways
- Human development is a genetically regulated process.
- The unfolding of the developmental pattern is referred to as the long-term genetic regulation.
- Genetic determinants that set the initial regulatory stage in an early embryo (master genes) are expressed in the mother and their mRNA is deposited to the oocyte.