Chapter 12. Genetics of Immunity
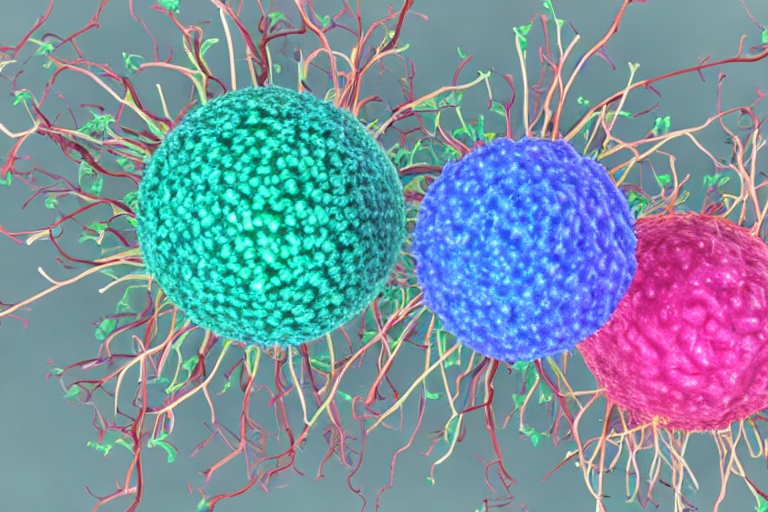
The Importance of Cell Surfaces
Foreign antigens (molecules) elicit an immune response from a host.
Antibodies and cytokines produced by the immune system attack foreign antigens.
Blood Groups
Early blood transfusions were oftentimes unsuccessful. With the discovery of human blood groups systems, properly matched donor and recipient types resulted in successful transfusions.
Blood types, including the ABO groups (Chapter 2), Rh factor, and others, result from self antigen patterns on red blood cells. Rh incompatibility may put a fetus at risk.
The Human Leukocyte Antigens
In addition to blood group antigens, a large number of cell surface molecules are recognized by the immune system.
Many of the cell surface proteins that help to establish immunity are encoded by the approximately seventy genes of the major histocompatibility complex (MHC) located on chromosome 6 in humans.
Class I and II genes of the MHC encode human leukocyte antigens (HLA).
Class III genes produce plasma proteins involved in the immune response.
HLA antigens on leukocytes are involved in processing of foreign antigens.
HLA proteins are each encoded by several genes with many alleles and as a result only 2 in every 20,000 unrelated people match for the six major HLA genes by chance.
Individuals with certain HLA combinations have an increased risk of developing certain HLA-linked diseases.
The Immune System
At the cellular level the immune system consists of various types of lymphocytes and macrophages.
The immune response consists of an immediate, generalized, innate immunity and a slower, more specific adaptive immunity.
Physical Barriers and the Innate Immune Response
Skin, mucous membranes, tears, and saliva are examples of physical and chemical barriers that keep pathogens from entering the body.
Pathogens that breach this barrier encounter an innate immune response consisting of inflammation, phagocytosis, complement, collectins, and cytokines.
The Adaptive (Acquired) Immune Response
The innate response is rapid (minutes) while the acquired immune response must be stimulated to action and takes days to respond.
The acquired immune response is divided into the humoral and cellular immune responses. Both of these responses differ from the innate immune response in that they are more complex, highly specific and each has a cellular component with memory.
The humoral response involves B cells that secrete antibodies in order to neutralize, clump, and stimulate the destruction of pathogens by recognizing and binding specific foreign antigens.
Antibodies are made of Y-shaped polypeptides consisting of constant and variable regions.
The astounding diversity of antibody binding activities is due to a shuffling of gene pieces (exons) encoding antibody polypeptide products in B cells.
In the cellular immune response, helper T cells stimulate B cells to manufacture antibodies and cytotoxic T cells to secrete cytokines.
Some T cells bind to nonself cells and virus-covered cells and burst them. Other T cells function to coordinate the immune response.
Abnormal Immunity Inherited Immune Deficiencies
Inherited immune deficiencies represent defects in the genes that encode proteins involved in immunity.
Acquired Immune Deficiency Syndrome
Acquired immune deficiency syndrome is caused by HIV.
HIV replicates very rapidly, and T cell production matches it until the immune system is overwhelmed and AIDS begins.
HIV is a retrovirus that injects its RNA into host cells by binding receptors. Reverse transcriptase then copies viral RNA into DNA. HIV uses the cell’s protein synthesis machinery to mass produce itself; then the cell bursts, releasing virus.
Reverse transcriptase and protease inhibitors have been effective in slowing down HIV.
Autoimmunity
In autoimmune disorders, autoantibodies attack healthy tissue. These conditions may be caused by a virus that borrows a self antigen, T cells that never learn to recognize self, or healthy cells bearing antigens that resemble nonself antigens.
Some conditions thought to be autoimmune may actually reflect an immune system response to retained fetal cells.
Mutations in some genes may present the symptoms of an autoimmune disease.
Allergies
An overly sensitive immune system causes allergies.
In an allergic reaction, allergens bind to IgE antibodies on mast cells, which release allergy mediators.
A subset of helper T cells secrete cytokines that contribute to allergy symptoms.
Altering Immune Function Vaccines
Vaccines are disabled pathogens or their parts that elicit an immune response, protecting against infection by the active pathogen.
Immunotherapy
Immunotherapy uses immune system components to fight disease.
Hybridomas are artificial cells that consist of a B cell fused with a cancer cell and produce monoclonal antibodies (Mabs) that can target specific antigens.
Cytokines boost immune function and destroy cancer cells.
Transplantation
Autografts transfer tissue from one part of a person’s body to another.
Isografts are tissue transfers between identical twins.
Allografts involve tissue transfers between members of the same species. These transplants can cause tissue rejection re- actions.
A Xenograft is a cross species transplant. A danger of these transplants is that they can set off a hyperacute rejection.
Graft-versus-host disease involves a rejection of recipient tissues by transplanted bone marrow.
The success rate of transplants is improved by the use of immunosuppressive drugs, by stripping antigens from donor tissue, and by matching donor to the recipient.
A Genomic View of Immunity – The Pathogen’s Perspective
Analyzing the genomes of pathogens may reveal the molecular basis of pathogenesis.
Crowd Diseases
Crowd diseases readily spread through populations and can cause epidemics.
Native populations with no immunity can be devastated by introduction of new diseases.
Bioweapons
Microorganisms like viruses, bacteria or fungi, and the toxic substances produced by them and other living organisms, can me modified and enhanced to produce agents that, when released deliberately, cause catastrophic disease and death in humans, as well as other living things. A variety of pathogens have been adapted to military use in the USA, the former Soviet Union, and other countries around the world. Biological agents like anthrax, botulinum toxin and bubonic plague can produce a public health emergency causing large numbers of deaths in a short amount of time. Biological agents which are capable of secondary transmission can lead to epidemics. An attack involving a biological agent may mimic a natural event, which may complicate the public health assessment and response.
Between the 1950-1980s, the Soviet Union had developed a wide range of weaponized agents, based on genetically modified agents, capable of causing devastating pandemics. Among these were antibiotic-resistant strains of plague, anthrax (called Siberian Ulcer in Russian), tularemia, and glanders. The full extent of the capabilities of Soviet bioweapons is not known. The Russian Federation that inherited the Soviet bioweapon stockpile, has the potential to use these agents, considering the reckless political behavior of the Russian state, particularly towards its immediate neighbors such as Ukraine, as well as the world at large.
The Epidemiology of the COVID-19 coronavirus infection
The severe acute respiratory syndrome coronavirus 2 (SARS-CoV-2) virus, better known as COVID-19-causing virus, or just COVID-19, emerged at the end of 2019 from a yet unidentified source, potentially in the city of Wuhan, China, causing a crowd infection that spread rapidly across the world. In the absence of verifiable source of the COVID-19 infection, COVID-19 can also be considered a potential bioweapon. By March 2020, COVID-19 cased a global pandemic. According to the World Health Organization (WHO), as of May 2023 one tenth of the planet’s population contracted COVID-19 and there have been nearly 7 million deaths from COVID-19 worldwide.
SARS-CoV-2 belongs to the coronavirus family of viruses and is related to the severe acute respiratory syndrome (SARS) and Middle East respiratory syndrome (MERS) coronaviruses. The COVID -19 virus uses the human ACE2 receptor to infect epithelial cells in the nasopharynx, where it replicates and subsequently enters the distal alveolar space. Human immune system recognizes the virus through cell receptors, primarily of the Toll-like receptor group such as TLR3 and TLR7, which induce the synthesis of type I interferons.
Individual response to the COVID-19 infection varies significantly among people. For some, the infection is asymptomatic, while other suffer symptoms varying in strength and proliferation. In 2020, several studies attempted to identify the genetic foundation of COVID-19 response. Several regions on chromosome 3 and 9 have been linked with the severe COVID-19 response. A further investigation determined that the regions associated with severe COVID-19 response on chromosome 3 appear to display high Linkage Disequilibrium (LD) (Zeberg, Pääbo, 2020). This means that these regions are highly associated with each other in the genome, resisting being separated by genetic recombination, which tends to dissociate linked genes (such as genes on the same chromosome), unless strong selective pressure favors these genes to remain linked. It was further determined that these linked genetic segments introgressed into human genome from Neanderthals (more on genomic introgression between humans and Neanderthals in Chapter 16).
It was determined that European and Siberian Neanderthals had some of these genetic sequences in homozygous state in their genome, and these sequences were absent in the Denisovans, a sister hominin group to Neanderthals (see Chapter 16 for a discussion on human extinct relatives). It was further determined that these sequences are unlikely to have existed in the common ancestor of humans and Neanderthals and that their appearance in the human genome was likely due to a an interbreeding event between the two hominin groups.
Of the human populations living today, the highest rate of the severe COVID-19 risk haplotype is registered in South Asia, particularly in Bangladesh, where more than half of the population carries these Neanderthal genetic variants. Surprisingly, the risk alleles are nearly absent in East Asian populations. The reason for these differences in the risk allele frequency between the two parts of the same continent is not entirely clear. In all likelihood, this is the result of a strong selection event in the past, except that many epidemiologists consider COVD-19 to be a novel virus to humans, making human encounters with COVID-19 prior to 2019 unlikely. There has to be something other than the response to COVID-19 in these genomic sequences that induced a strong selective response in the past.
A closer look at the genomic region on chromosome 3 associated with the COVID-19 risk haplotype reveals that the region harbors several genes vital to human immune response, specifically, the CCR gene cluster.
The CCR gene cluster encodes members of the beta chemokine receptor family. The ligands of these receptors include macrophage inflammatory protein 1 alpha (MIP-1 alpha), regulated on activation normal T expressed and secreted protein (RANTES), monocyte chemoattractant protein 3 (MCP-3), and myeloid progenitor inhibitory factor-1 (MPIF-1). Chemokines and their receptors mediated signal transduction are critical for the recruitment of effector immune cells to the site of inflammation. Knockout studies of the mouse homolog suggested the roles of this gene in host protection from inflammatory response, and susceptibility to virus and parasite. The chemokine receptor genes, including CCR2, CCRL2, CCR3, CCR5, CCR9 and CCXCR1, are found to form a gene cluster on chromosome 3p.
The COVID 19-associated region contains the CCR9 gene. CCR9 is differentially expressed by T lymphocytes of small intestine and colon, suggesting a role in the thymocytes recruitment and development that may permit functional specialization of immune responses in different segment of the gastrointestinal tract.
The rs35044562 (A-G) single nucleotide polymorphism (SNP), which is at strong LD with 12 other SNPs within the COVID 19 risk region in non-African populations, is at the very beginning of the COVID- 19 associated region on chromosome 3. The same region harbors the gene called the LZTFL1 transcription factor (when read on the opposite DNA strand in the 5′-3′ direction). LZTFL1 in a putative tumor suppressor region on chromosome 3. The COVID 19 risk haplotype is almost entirely within the LZTFL1 gene.
It is easy to imagine that the combination of CCR9 and LZTFL1 would be vitally important to modern humans as they ventured out of Africa into Eurasia (see Chapter 16). Thus, it should be of no surprise that a chromosomal region carrying an immunity-boosting and cancer-suppressing gene sequence introgressed from the locally adapted Neanderthals as humans were making their way into uncharted new territory would be a vital aid to survival and would be strongly favored by natural selection. It is likely an unfortunate coincidence that this same region would confer a severe response to a novel viral infection. At the same time, this response may be reflecting a discrete evolutionary adaptation whereby the immune response genes being adapted to known viruses produce an overwhelming and ultimately negative response to a new viral agent.
Decoding the Genetics Behind COVID-19 Infection
Researchers join forces to unravel a biological mystery
February 17, 2022
https://covid19.nih.gov/news-and-stories/decoding-genetics-behind-covid19-infection
The repost of the article adheres to the Fair Use application of 17 U.S. Code § 107.
Since the start of the pandemic, nearly everyone has wanted to know the answer to one question: What is responsible for the different ways people’s bodies respond to SARS-CoV-2 infection? Some people infected with SARS-CoV-2, the virus that causes COVID-19, are asymptomatic; some develop moderate but manageable COVID-19; and some, including individuals who are young and otherwise healthy, become severely ill or die from the disease. Some people may never test positive for COVID-19, even while living with an infected person.
Scientists think that for some people the answer lies in the genes, the blueprints we inherit from our parents that play a role in determining everything from our eye color to our risk of having a heart attack. Our genes also play a role in how our immune system responds to invaders and the characteristics of our lung cells, which can be attacked during the early stages of SARS-CoV-2 infection.
In two studies supported in part by the National Institute of Allergy and Infectious Diseases (NIAID) and the National Human Genome Research Institute (NHGRI), researchers working separately with COVID-19 patients and human lung cells are studying the genetics behind why people are less or more likely to develop COVID-19. These scientists have made unprecedented progress in record time and are laying the groundwork for drug and vaccine developers to create new treatments and vaccines, as well as improve existing ones.
A Misguided Immune Response to COVID-19
“In 18 months, we’ve been able to determine that genetics account for nearly 20% of critical cases of COVID-19, including pneumonia,” said Jean-Laurent Casanova, M.D., Ph.D., of the Rockefeller Institute and the Howard Hughes Medical Institute. His research is funded by NIAID.
Casanova co-leads the COVID Human Genetic Effort, a collaboration of more than 400 researchers and 40 sequencing hubs in 50 countries. He and his collaborators sequenced the genomes of thousands of patients with life-threatening COVID-19, publishing four papers detailing genetic similarities between people hospitalized with critical COVID-19 pneumonia.
The group found that, in some cases, the immune system mistakenly flagged and q attacked proteins called type I interferons (IFNs) that help regulate immune response. Normally, antibodies attach to foreign invaders, marking them for destruction by other immune cells. But the researchers discovered that some people made “auto-antibodies,” antibodies against their own type I IFNs. Nearly 20% of the people who died from COVID-19 created auto-antibodies.
“Their immune systems mistakenly depleted their IFNs, making them more susceptible to the virus,” Casanova said. These IFN-targeting auto-antibodies are found in uninfected individuals too, suggesting that they may contribute to the development of severe COVID-19. “Critical COVID-19 pneumonia probably results from insufficient type I IFNs in the first days of infection,” Casanova said.
Finding the Genes That Create Resistance to COVID-19
Neville Sanjana, Ph.D., of the New York Genome Center and New York University, was interested in another part of the question: What genes protect cells from SARS-CoV-2 infection? With support in part from NHGRI, Sanjana and his colleagues used the gene editing tool CRISPR to figure out which host genes were essential to viral infection, and to what degree. The team used CRISPR to disable each individual gene that makes up the human genome in human lung cells. Lungs are known to be the primary site of infection for SARS-CoV-2, though other organs and tissues are also affected.
With the help of Benjamin tenOever, Ph.D., of New York University, the researchers then exposed the cells to SARS-CoV-2. Disabling most of the genes didn’t make a difference, and the cells died when infected with SARS-CoV-2. But some genes seemed to help SARS-CoV-2 infect cells; when those genes were disabled, most of the cells survived the infection.
The researchers then ranked every gene by how easily SARS-CoV-2 was able to infect host cells after the gene was inactivated. The gene that codes for a protein on the surface of lung cells, known as the ACE-2 receptor, ranked eighth out of 20,000 genes, confirming for Sanjana and his colleagues that their CRISPR assay could pinpoint essential genes for infection. The ACE-2 receptor was already known to be required for SARS-CoV-2 infection, because SARS-CoV-2 needs to bind to it before entering the cell.
Their experiment confirmed that several genes that were already suspected are important for SARS-CoV-2 infection, but the team also found other genes had undiscovered roles. And those genes were active beyond the lung, possibly helping explain how the virus can spread beyond the lungs to other organs. “Some of the key human genes that the virus seems to depend on for successful infection are actually broadly expressed throughout the body. And, as we have seen, once it gets in the lung, the virus seems to spread to other tissues in people with severe infections,” Sanjana said.
Sanjana and his colleagues did not want to stop there. They searched for existing drugs that might be used to inhibit the top-ranked genes and tested whether those drugs blocked SARS-CoV-2 infection. Several drugs appeared to do just that, including amlodipine, a medication used to treat high blood pressure. Moving forward, scientists can use this research in their search for promising COVID-19 treatments.
The Unpredictability of Emerging Variants
These research groups continue to work at an unprecedented pace, given the unpredictability of emerging SARS-CoV-2 variants. For example, Sanjana and colleagues are developing tests to understand the mechanisms that enable new variants to become more infectious. They showed how one of the first spike protein mutations, D614G, allowed SARS-CoV-2 variants to enter host cells more easily than the original viral strain could. Today, all currently circulating strains of SARS-CoV-2, including Delta and Omicron, carry the D614G mutation. The Sanjana lab is also developing new genome editing tools to explore the interactions between specific human genetic mutations and viral variants.
For many scientists, the pandemic has led to an “all-hands-on-deck” response. The scientific community has shown that it can work together in new ways, step outside of usual specialties, produce rapid results, and influence the course of the pandemic. “As basic geneticists,” Sanjana said, “we rarely get the chance to do this kind of translational work where we can literally hand off drug candidates to drug developers around the world. It’s wonderful to see how people have picked up different aspects of the work and are running with it.”
Key Takeaways
- The complexity of human immune response is the result of a flexible “modular” arrangement of genes that regulate immunity.
- Modern humans inherited some of the key immune responses genes from extinct hominins such as Neanderthals.