Chapter 1. The Science of Genetics: An Overview
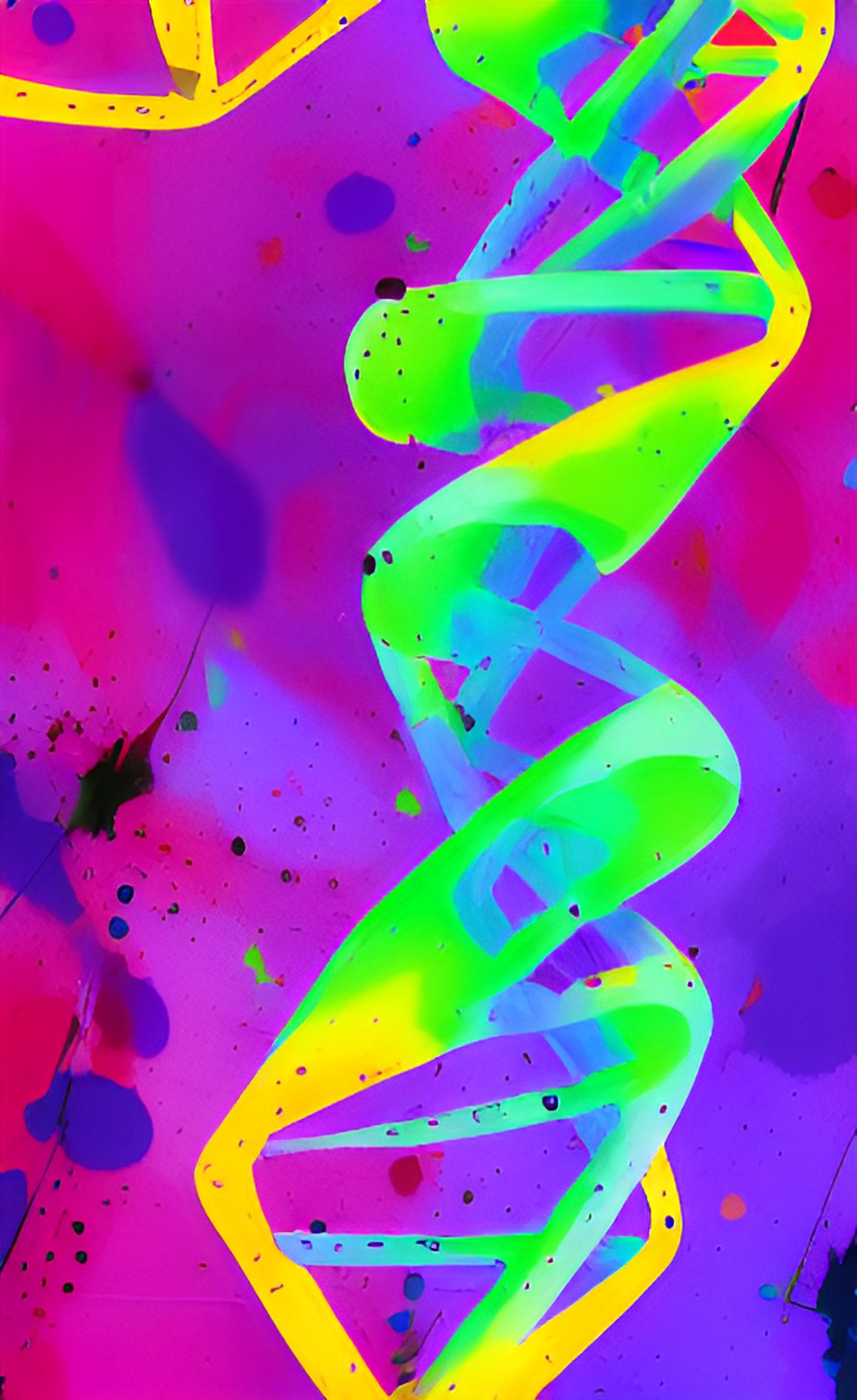
The subject of Genetics
Genetics is the study of inheritance and variation. Transmission Genetics studies mechanisms of gene transmission from generation to generation. Molecular Genetics studies the molecular basis of inheritance (structure and function of genetic material at the molecular level). Biotechnology (Genetic Engineering) is applied molecular genetics. Cytogenetics studies chromosomal structure and function. Population Genetics studies genetic variation in groups of organisms.
Human Genetics is the study of inheritance and variation in human beings. Medical Genetics is the study of medical aspects of human genetics.
The principles of inheritance in humans do not fundamentally differ from that in other living organisms. An understanding of human genetic inheritance is a vital asset in the diagnosis, prediction, and treatment of medical conditions that have a genetic foundation.
DNA and human cells
The human body is made up of 100 trillion cells. Each cell (except red blood cells) has at least one nucleus, which houses the chromosomes.
There is 1.8 m of DNA in each of our cells packed into a structure only 0.0001 cm across.
Most human somatic cells (cells of the body) contain 46 chromosomes: pairs of chromosomes 1-22 (autosomes), and a pair of sex chromosomes.
Females normally have two X chromosomes; males have an X and a Y.
Human reproductive cells (sperm cells and oocytes) contain one set of 22 autosomes and one of each sex chromosomes.
The ABC of GATC: The chemical organization of nucleic acids
Deoxyribonucleic acid (DNA) and ribonucleic acid (RNA) comprise the nucleic acid family of bioorganic molecules. Besides nucleic acids, bioorganic molecular groups include proteins, carbohydrates and lipids.
DNA and RNA are biopolymers, meaning that they are formed from connecting together simple building blocks called monomers. In the case of DNA and RNA, the monomers are called nucleotides.
Nucleotides themselves consist of a pentose monosaccharide called ribose (in RNA) and deoxyribose (in DNA), a phosphate functional group and a compound called nitrogenous base. Deoxyribose is derived from ribose by the loss of an oxygen atom in the hydroxyl group connected to the second (2’) carbon of the pentose molecule. There are five types of nitrogenous bases found in nucleic acids. Three of the five (adenine, guanine, cytosine) are found in both DNA and RNA, while thymine is only found in DNA and uracil is only found in RNA.
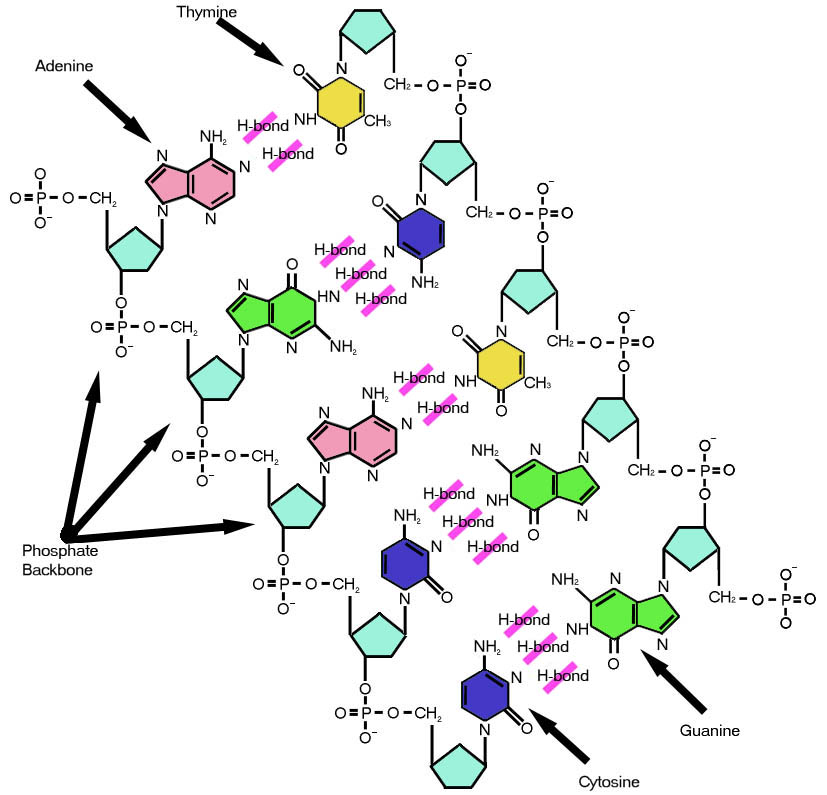
The Human Genome
The Human Genome Project (HGP) is an endeavor that could be compared in complexity and significance to a trip to Mars. If you were to read the entire genome of a human being, like it were a book, one base at a time, it would take you 140 years to complete! By 1985, when serious talks about the sequencing of the human genome began to develop, Jim Watson, first HGP Director (1988 to 1992), estimated that it would take 1,000 years to complete with the existing technology at the time. In October of 1990, when the HGP was officially launched, it was intended to be a 20-year project, where most of the time would be devoted to improving of technology, rather than actual sequencing. In 1997, the estimate was that it would take another 50 years to actually complete the sequencing. Despite the prognosis, the first “rough draft” of the human genome was completed in 2000, at which point it was expected that the mapping of the genome would be completed in five years. The essentially completed human genome assembly was released in April 2003, 2 years earlier than planned.
Why is the knowledge of the human genome important?
The knowledge we have gained from the deciphering of the human genome has significantly impacted modern medicine. Doctors can now tailor medications to your specific genetic makeup. Pharmacogenetics, the field of medicine concerned with how your genes affect your response to drugs, can now find ways to reduce side effects and improve drug efficiency, by taking into consideration how your genes may react to medications you are prescribed. Other benefits include being able to anticipate the probability of developing cancer based on an individual genetic makeup as well as being able to predict the specific genetic conditions children of perspective parents may have. The are countless other benefits, which we will be discussing throughout the course.
The Human Genome At A Glance
Total number of chromosomes: 46
Haploid genome: 23 chromosomes
Molecular size: 3.3 billion (3,323,950,079) nucleotides (or base pairs, bp)
Largest chromosome #1 = ~263 million base pairs (bp)
Smallest chromosome Y = ~59 million bp
Known protein-coding genes: 19,940 (GENCODE reference genome version 29, 2018)
RNA genes (regulatory, tRNA, rRNA etc.): 23,643
Pseudogenes: 14,729
Gene transcripts (detected mRNA): 206,694
Protein-coding transcripts: 83,129
Mobile genetic elements: 45% of the genome
Average size of a human gene: ~3,000 bp
Only about 5% of the human genome contains genes (coding sequences)
Less than 2% of the genome codes for proteins
Humans share most of the same protein families with worms, flies, and plants
Function of much of the genome is unknown
At least 20% of the non protein-coding portion of the genome is dedicated to regulating the transcriptional activity of the encoding 2%.
Repeated sequences that do not code for proteins make up at least 50% of the human genome. Recent studies suggest that that 66%–69% of the human genome is repetitive or repeat-derived (de Koning et al., 2011)
45% of these repeated sequences are able to move around within the genome (mobile genetic elements, or transposable elements)
Below is a link to a map of human chromosomes and associated genetic diseases:
http://www.ncbi.nlm.nih.gov/books/NBK22266/
Mobile genetic elements
Mobile genetic elements (MGEs), also referred to as transposable elements (TEs) or transposons, are nucleotide sequences normally housed within the genome that retain the ability to change their location. Transposons are classified into retroelements (Class I MGEs) and DNA transposons (class II MGEs). The latter are sometimes referred to as “jumping genes” or transposable elements, since the movement of transposons requires the presence of a functional enzyme called transposase. Retroelements can be transcribed from DNA to RNA and the RNA produced is then reverse transcribed to DNA. Reverse transcription is accomplished with the help of an enzyme called reverse transcriptase (RNA dependent DNA polymerase), sometimes encoded by retroelements themselves. This copied DNA is then inserted at a new position into the genome. Transposons have a tendency to re-insert into the non-coding regions of the genome, but they can also insert in the vicinity of functional genes, thereby interrupting vital functions. DNA transposons comprise 8% of nuclear genome. MGEs are found on all chromosomes, but mostly on the X and chromosome 19. More on transposons in Chapter 10 (Mutations).
Comparative Genetics and its Importance
Basic assumption of genetic research: similar mechanisms control genetic processes in different organisms.
By studying the genetic mechanisms in simpler genetic models, we will be able to uncover basic principles of genetic inheritance.
Using the knowledge of genetics gained in the study of model organisms, we will be able to understand the mechanisms of genetic inheritance in human beings.
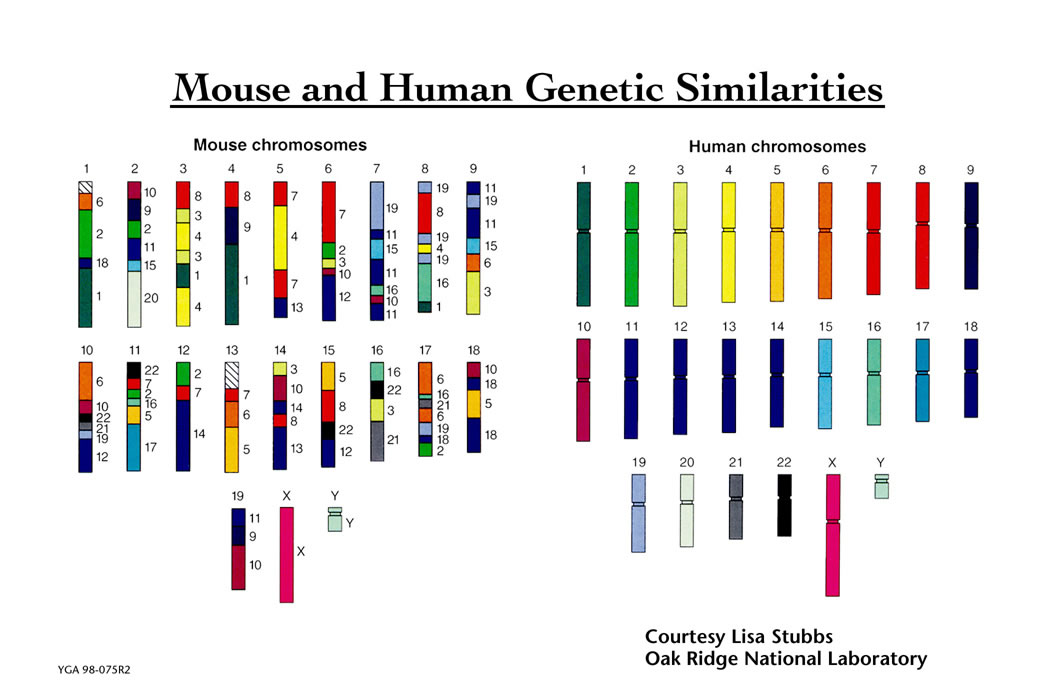
This figure demonstrates the genetic similarity (homology) of the superficially dissimilar mouse and human species. The similarity is such that human chromosomes can be cut (schematically at least) into about 150 pieces (only about 100 are large enough to appear here), then reassembled into a reasonable approximation of the mouse genome. The colors and corresponding numbers on the mouse chromosomes indicate the human chromosomes containing homologous segments.
Favorite model organisms of geneticists
E. coli (4,377 genes, one chromosome)
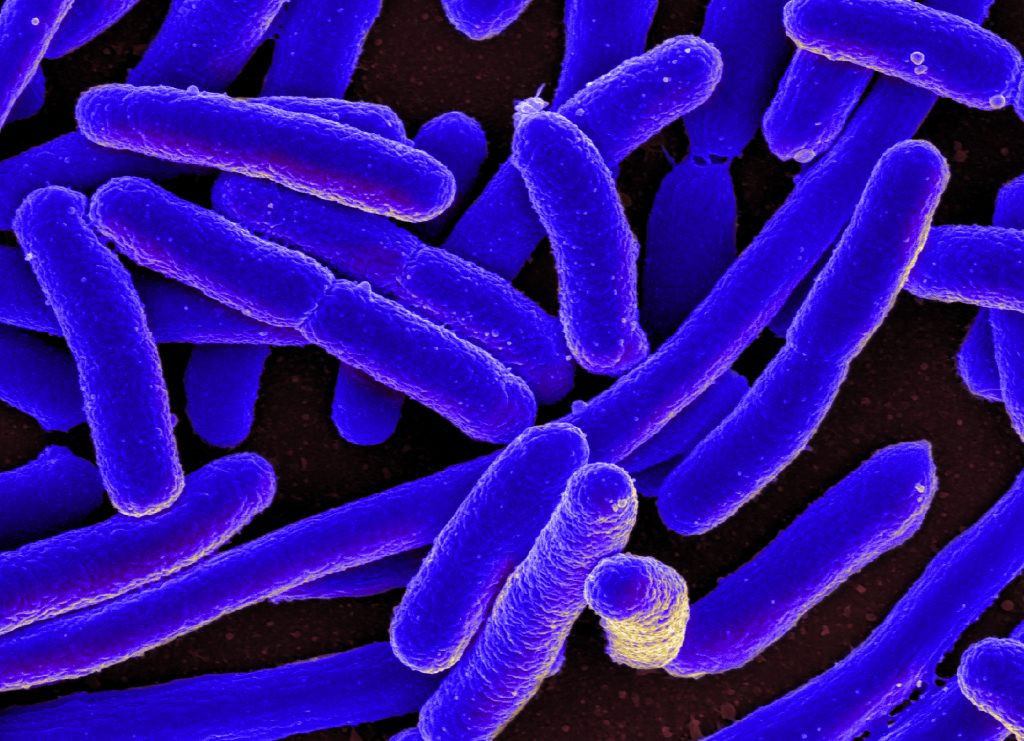
Neurospora sp. (~10,000 genes, 14 chromosomes)
C. elegans (~20,000 genes, 12 chromosomes)
Drosophila sp. (~14,000 genes, 8 chromosomes)
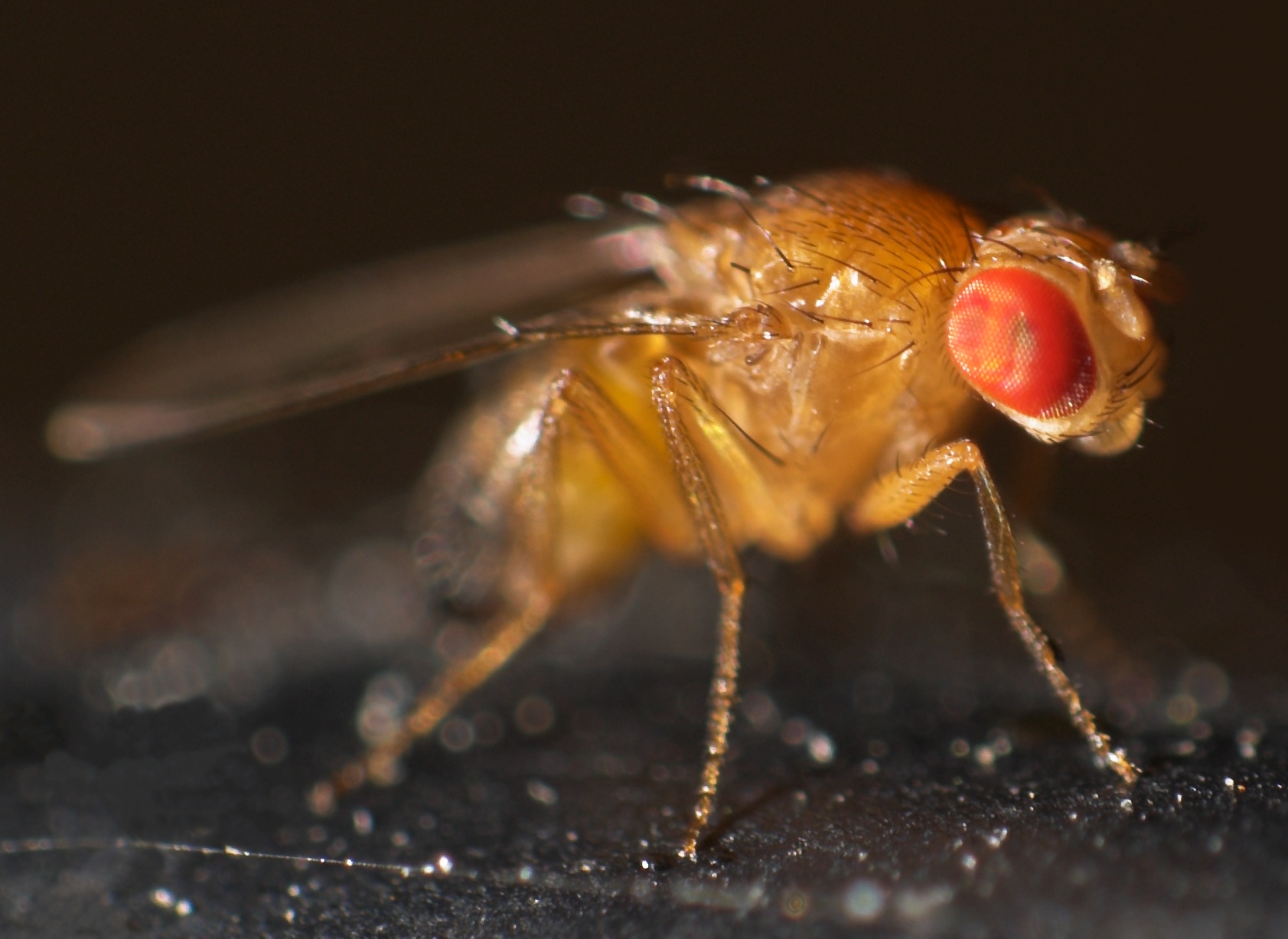
Mus musculus (3 billion bp, 23,786 genes, 40 chromosomes)
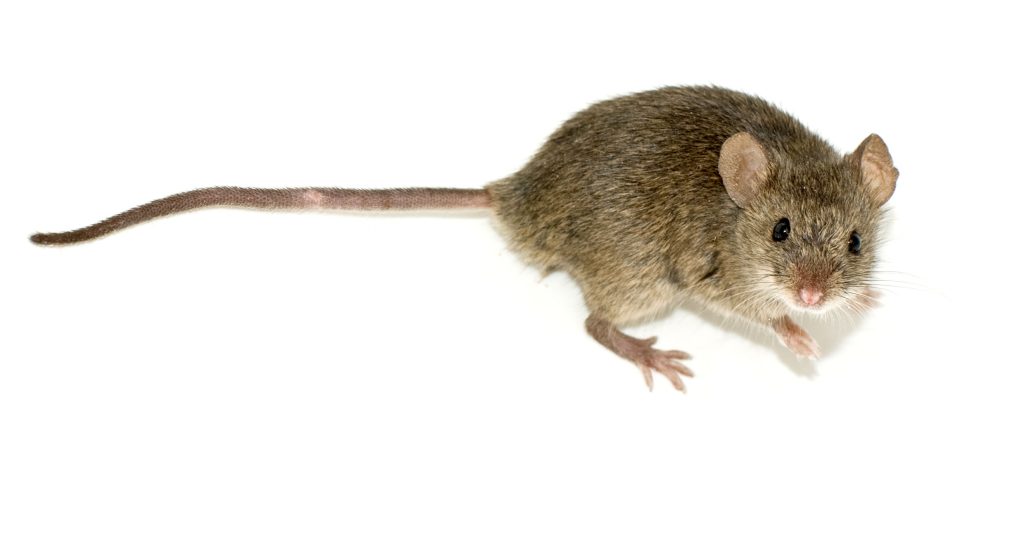
A Brief Overview of the Scientific Method
Genetics, like any other field of science, deals with observable and measurable phenomena.
The Scientific Method is a thought process that scientists use to produce a comprehensive and objective (i.e., unbiased, not dependent on an individual opinion) explanation of the processes that govern natural world. Scientific knowledge doesn’t have a value system; it generates and tests hypotheses based upon observations.
The primary goal of the Scientific Method is to acquire knowledge and understanding about the natural world through systematic investigation. It aims to discover patterns, establish causal relationships, and develop theories or models that can explain and predict phenomena. The scientific method strives for objectivity and aims to generate reliable and valid results that can be tested and replicated by others.
The role of Parsimony in the scientific analysis
“Pluralitas non est ponenda sine neccesitate”, plurality is not to be posited without necessity (Parsimony Rule/ Ockham’s Razor).
“Of the two competing explanations, both of which are consistent with the observed facts, we regard it as right and obligatory to prefer the simpler” (Barker, 1961, p. 273).
Parsimony, also known as the principle of simplicity or Occam’s razor, plays a vital role in scientific analysis. It suggests that among competing explanations or hypotheses, the simplest one (or, rather, the one that takes the fewest steps to explain the phenomenon in question) that can account for the observed data should be preferred until further evidence is available.
In scientific analysis, parsimony serves as a guiding principle for selecting the most likely explanation or hypothesis that requires the fewest assumptions or complexities. Here are a few ways parsimony contributes to scientific analysis:
Parsimony, also known as the principle of simplicity or Occam’s razor, plays an important role in scientific analysis. It suggests that among competing explanations or hypotheses, the simplest one that can account for the observed data should be preferred until further evidence is available.
In scientific analysis, parsimony serves as a guiding principle for selecting the most likely explanation or hypothesis that requires the fewest assumptions or complexities. Here are a few ways parsimony contributes to scientific analysis:
- Simplicity and Explanation: Parsimony encourages scientists to favor explanations that require the fewest assumptions or entities. It suggests that simpler explanations are more likely to be true or accurate. By choosing simpler hypotheses, scientists aim to provide concise and elegant explanations for observed phenomena.
- Occam’s Razor: Occam’s razor is a principle related to parsimony, often attributed to the philosopher William of Ockham. It states that “entities should not be multiplied beyond necessity.” In scientific analysis, Occam’s razor advises against introducing unnecessary or extravagant elements when simpler explanations are available.
- Testability and Predictions: Simpler hypotheses are often easier to test and make specific predictions. They provide clearer expectations that can be compared with empirical data. Parsimony helps scientists avoid excessive complexity, ensuring that hypotheses can be tested and evaluated effectively.
- Model Selection: In fields where multiple models or theories exist to explain the same phenomenon, parsimony can guide scientists in selecting the most appropriate one. By favoring simpler models, scientists aim to avoid overfitting the data, reduce complexity, and enhance the model’s generalizability.
- Communication and Clarity: Parsimony helps scientists communicate their findings more effectively. Simple explanations and models are often easier to understand and convey to others, enhancing clarity and accessibility in scientific communication.
It is important to note that while parsimony is a valuable principle in scientific analysis, it is not a definitive rule. In certain cases, complex explanations may be necessary to account for all available evidence. Ultimately, the principle of parsimony should be balanced with the need to adequately explain and account for the complexities of the natural world.
Steps of the scientific inquiry
The Scientific Method is a systematic approach used by scientists to investigate and acquire knowledge about the natural world. It provides a structured process for conducting scientific research and includes several key steps:
- Observation and description of a phenomenon.
- Formulation of a tentative explanation for the observed phenomenon (formulation of a hypothesis).
- Making predictions based on the hypothesis.
- Testing the predictions by conducting a series of experiments.
- If the results of the tests support the predictions, the hypothesis becomes a theory.
- The theory becomes generally accepted when different research groups perform independent tests of the theory and obtain comparable results.
It should be kept in mind that the Scientific Method is an iterative process, and scientific knowledge is constantly revised and refined based on new evidence and insights.
Science look-alikes
Beware of what’s called “science by press conference,” when scientists announce their results to the media, before they’ve been reviewed by their peers and published in scientific journals. An example of such activity is the announcement in November of 2001 of the first successful attempt to clone a human embryo made by Dr. West, the director of the Advanced Cell Technology (ACT) company in MA, which was made at a press-conference, before other scientists in the field got a chance to take a look at it. Upon closer examination by peers, it appeared that the achievement wasn’t so great, the clone didn’t survive past the third cell division, but the media turned this premature scientific announcement into a sensation and the procedure drew sharp criticism, followed by health policy changes, from the US Government. Thus, to the public (and the government) it appeared as though the scientists succeeded in cloning a human being.
Key Takeaways
- Human Genetics is a branch of Genetics concerning the principles of inheritance in human beings.
- The principles of Human Genetics are not fundamentally different from the principles of Genetics that govern the inheritance in any other living species.
- Comparative genetics studies the principles of genetic inheritance using model systems.
- Genetics is governed by the principles of the Scientific Method inquiry.